Dr. Burgess received his Ph.D. in genetics from the Johns Hopkins University School of Medicine, where he studied the genetics of mitochondrial fusion and fission in yeast. He trained with Nancy Hopkins, Ph.D., at the Massachusetts Institute of Technology, where he was part of a large effort to develop insertional mutagenesis in zebrafish, coupled with a genetic screen to identify genes essential for early development of a vertebrate. Since 2001, he has been at the National Human Genome Research Institute, where he is a senior investigator and heads the Developmental Genomics Section.
Much of Dr. Burgess' work in the last decade has been focused on developing efficient gene knockout technologies in zebrafish, coupled with efficient phenotyping of the gene disruptions.
The completion of the Human Genome Project gave us an unprecedented view of the organization of a vertebrate genome, but it also raised as many questions as it answered. One key discovery was that it took approximately 20,000 genes to make a human being. However, for more than 14,000 of those genes, the function has never been tested in any organism. This becomes a significant problem when researchers are attempting to show a link between a specific gene and a biological trait or a human disease. For more than a decade, the focus of the Developmental Genomics Section has been to develop techniques for high-throughput testing of gene function in vivo, using zebrafish as a vertebrate model system. Much of the lab effort revolves around systematically inactivating genes by mutation to see the resulting effect on the animal (i.e. the phenotype). Initially, this involved causing random lesions in the genome and identifying when the lesions inactivated genes. These mutant fish were cryopreserved to create a library of gene knockouts in zebrafish. Newer technologies have enabled a shift effort towards directed gene knockouts using genomic editing via targeted nucleases.
Tissue regeneration is the result of a complex integration of injury signals, stem cell activation, inflammation responses, reactivation of developmental programs and regeneration-specific processes. We have developed a systematic approach to dissect and analyze the various pathways involved in hearing regeneration by using zebrafish as a model organism. By using a high-throughput “guided” genetics and chemical genomics screen to identify the key genes and pathways involved in hearing regeneration, we have significantly enriched our success rate for identifying regeneration-specific genes. From our previous work in collaboration with Dr. Popper, we had collected 2000 candidate genes involved in hearing regeneration by transcriptionally profiling regenerating zebrafish adult inner ears after sound damage. We’ve built an efficient gene knockout pipeline, first using retroviral mutagenesis but now using CRISPR/Cas9 targeting and we are systematically inactivating the 2000 candidate genes and testing their role in both normal hair cell development and hair cell regeneration.
In addition, we have screened a broad number of well-characterized pharmacological inhibitors to identify genes that cannot be easily tested by KO because of their important roles in early development. From our first one hundred and fifty genes and twenty chemical inhibitors tested, we have identified four genes that reduce the number of hair cells in a normal embryo, and we have identified an additional seven genes and three chemical inhibitors that specifically disrupt regeneration of the hair cells without affecting normal development. We will present data on the genetic strategy used to generate and screen hundreds of zebrafish gene knockouts for hearing regeneration defects, the pathways emerging from our genetic and chemical genomic analysis, and the deeper phenotypic characterization of a sample regeneration-specific mutant we have identified.
Current Students
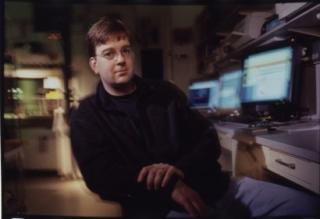