Personal Statement
Overall, the main goal of my research has been to investigate neural mechanisms underlying learning, executive control and decision-making, and their disturbance in addiction. Specifically, I record neural activity from various brain regions as rats perform a variety of cognitive tasks (e.g. reversal, delay discounting, stop-signal, set-shifting, conflict, social) and evaluate loss of function after pharmacological or optogenetic manipulation. I have nearly 25 years of experience performing, analyzing, and publishing on this type of research. I completed my dissertation work in the lab of Dr. Carl Olson in the Department of Neuroscience (CNUP) and the Center for the Neural Basis of Cognition (CNBC) at the University of Pittsburgh. There, I recorded from single neurons in several areas in primate frontal and medial cortex during performance of reward-based saccade tasks. After graduating, I accepted a position as a post-doctoral fellow on the Cellular and Integrative Neuroscience Post-Doctoral Training Grant at the University of Maryland Medical School under the advisement of Dr. Geoff Schoenbaum. There I continued my work on issues pertaining to value-guided decision-making. Most of these studies used a novel choice task that I developed in Dr. Schoenbaum’s lab. During my time at the School of Medicine, I complemented my skills as a behavioral electrophysiologist with the ability to conduct perturbation experiments in order to behaviorally validate neural correlates. I also acquired the skills necessary to examine the impact that cocaine, schizophrenia-like brain alterations (NVHL model), and age has on behavior, firing of single units and populations of neurons, and local field potentials. I have continued this NIH funded work as an assistant, associate, and full professor at the University of Maryland College Park as part of the Department of Psychology and as Director of the Program in Neuroscience and Cognitive Science, and have expanded my research to include processing of information in social paradigms. Further, we have adopted optogenetics and Fast-Scan Cyclic Voltammetry (FSCV) in the lab under the guidance of Joe Cheer as part of a seed grant awarded to us by the University of Maryland. Most recently, I have expanded my work to investigate the ability of molecular container compounds (MCCs) to bind and reverse the effects of multiple drugs of abuse, including PCP, methamphetamine and fentanyl in collaboration with Dr. Lyle Isaacs and Dr. Adam Brockett During the conduct of this research at the University of Maryland (14 years), I have mentored over 50 undergraduates, 6 graduate students and 4 post-docs. This includes 2 honors undergraduate research teams (11 students per team), 5 honors thesis students and 1 HHMI Fellow/ Fulbright Scholar. In 2013 and 2017 I received “Mentor of the Year” and “Outstanding Mentor” awards from the Honors College, in 2018 I received the “Excellence in Research Reward” from the college of Behavioral and Social Sciences and was named a “Distinguished Scholar-Teacher” by the University of Maryland in 2022.
Contributions to Science
In general, my research has focused on circuits critical for reinforcement learning, reward-guided decision-making and executive control, and how these circuits are disrupted in animal models of addiction, schizophrenia, and aging. Improving our understanding of the neural mechanisms underlying these functions has provided a better working knowledge of how we learn and behave normally, as well as what changes with mental disease and age. My contributions to the field are broken down into the following sections. I have 90 publications, 9500 citations and an h-index of 43 (Google Scholar).
https://scholar.google.com/citations?hl=en&user=Bwqic3IAAAAJ&pagesize=100&view_op=list_works
Encoding of Value and Motivation: I began my research career focusing on questions related to understanding what is genuinely signaled by neurons in the brain that fire strongly to environmental stimuli that predict more valued reward. During this time, several brain areas had been described as encoding the economic value of cues that predicted reward. These signals were thought to be important in guiding decisions based on anticipated outcomes. We contributed to this field of research by describing activity in several brain areas in frontal cortex that increased firing when monkeys were trained to expect a high versus low value reward. Surprisingly, we found that this value encoding (higher firing when the monkey was working for a better reward) was more prominent in areas strongly affiliated with the motor system (e.g., premotor cortex) compared to areas more strongly affiliated with the limbic/reward system (e.g., orbitofrontal cortex). This observation made us realize that “value encoding” might not genuinely reflect the value of the reward expected, but rather the motivated effort that the animal puts forth while working for more valued rewards. Indeed, monkeys performed the task faster and with higher accuracy when promised a more valued reward. To resolve this issue we designed a novel task in which we manipulated motivation independently from reward value. Consistent with our hypothesis, we found that reward-related activity in limbic and motor regions reflected two extremes of a continuum, one in orbitofrontal cortex that represented the value of the predicted reward, and another, manifested in premotor cortex that reflected the degree of motivation driven by the value of the reward. These results have called into question what information is actually encoded in many other brain areas reported to exhibit reward-related activity. This work continues to be an important focus of my research today and has expanded into questions pertaining to the processing of social information (see #5 contribution below for more information).
Roesch, M.R. and Olson, C.R. (2004) Neuronal Activity Related to Reward Value and Motivation in Primate Frontal Cortex. Science. Apr 9;304(5668):307-10
Bissonette, G.B., Burton, A.C., Gentry, R.N., Goldstein, B.L., Hearn, T.N., Barnett, B.R., Kashtelyan, V. & Roesch, M.R. (2013) Separate populations of neurons in ventral striatum encode value and motivation. PLoS One, 8, e64673.
Bissonette, GB, Gentry RN, Padmala S, Pessoa L, Roesch MR. Impact of appetitive and aversive outcomes on brain responses: linking the animal and human literatures. Frontiers in Systems Neuroscience. Front Syst Neurosci. 2014 Mar 4;8:24.
Gentry R, Lee B, Roesch, MR. Phasic dopamine release in the rat nucleus accumbens predicts approach and avoidance performance. Nature Communications. 7; 2016
Reward predictions, prediction errors and attention for learning: Since the beginning of my career I have carefully dissected encoding related to reward-guided decision-making and reinforcement learning across several brain areas. I developed a novel odor guided decision-making task that varies the value of reward by making the reward delivered at the end of each behavioral trial larger or more immediate across several trial blocks. This task has allowed us to address several issues related to encoding of reward prediction errors, reward predictions, attention, and different types of associative encoding that govern choice and instrumental behavior. This behavioral task has been very fruitful. To date, the task has been the topic of over 25 publications. With this behavioral paradigm we have shown that orbitofrontal cortex, anterior insula, and ventral striatum signal reward expectancies, and that dopamine neurons and neurons in basolateral amygdala signal signed and unsigned errors in reward prediction, respectively. Error signaling by dopamine neurons is dependent on input from orbitofrontal cortex and nucleus accumbens, and appears to represent theoretical signals put forth by Rescorla-Wagner. On the other hand, signals in basolateral amygdala seem to correspond to attentional signals proposed by Pearce-Hall, increasing firing to both unexpected reward and omission. We suspect that these signals inform mechanisms in anterior cingulate cortex to increase attention on trials after reward contingencies are violated so that learning can occur. Interestingly, our work also suggests that these two signals influence each other and do not entirely guide behavior through independent parallel pathways.
Roesch, M.R., Taylor, A.R. & Schoenbaum, G. (2006) Encoding of time-discounted rewards in orbitofrontal cortex is independent of value representation. Neuron 51, 509-20.
Roesch*, M.R., Calu*, D.J. and Schoenbaum G., (2007) Dopamine neurons in rat ventral tegmental area encode the more valuable option when deciding between differently sized and delayed rewards. Nat Neurosci: Dec;10(12):1615-24.
Bryden DW, Johnson EE, Tobia SC, Kashtelyan V, Roesch MR. (2011) Attention for learning signals in anterior cingulate cortex. J Neurosci. Dec 14;31(50):18266-74.
Lee B, Gentry RN, Bissonette GB, Herman RJ, Mallon JJ, Bryden DW, Calu DJ, Schoenbaum G, Coutureau E, Marchand AR, Khamassi M, Roesch MR. (2018) Manipulating the revision of reward value during the intertrial interval increases sign tracking and dopamine release. PLoS Biology. Sep 26;16(9):e2004015.
Animal models of addiction, schizophrenia, and aging: When I began examining animal models of drug use it was known that addicts and drug-exposed animals had decision-making deficits in reversal-learning tasks and more complex ‘gambling’ variants that require flexible behavior. We furthered this field by showing that cocaine-exposed rats were hypersensitive to changes in expected reward size and delay to reward. Although these behavioral deficits were well known, it was still unclear what neural signals were affected. Across several publications, we showed that chronic cocaine exposure impacted several nodes in the circuit critical to these types of behaviors. We demonstrated that flexible encoding of outcomes during decision-making in orbitofrontal cortex and basolateral amygdala was impaired after drug exposure. This miscoding was critical to the expression of the reversal-learning deficit, as demonstrated by ‘fixing’ the impaired behavior of cocaine-treated animals by making lesions to amygdala. In a different experiment, we showed that cocaine-exposure also reduced the degree and flexibility of cue-evoked firing in ventral striatum and anterior insula while enhancing cue-evoked firing in dorsal striatum, consistent with the idea that long term drug exposure makes behavior more habit-like. More recently, we have shown that lesions to ventral striatum can also enhance stimulus-response encoding in dorsal striatum, and that prior cocaine self-administration impairs attention and reward signals in anterior cingulate cortex and nucleus accumbens, respectively, and shifts encoding in dorsal striatum away from representing the action that will be taken to achieve a desired outcome. Together, these results have provided neurophysiological evidence that exposure to cocaine can cause behaviorally relevant changes in the processing of associative information, which is critical for the decline in behavioral flexibility observed after chronic drug exposure. In addition to the impact that drugs of abuse have on this circuitry, we examine how age disrupts brain and behavior. Specifically, we have shown that age reduces attention for learning signals and reward-related signals in amygdala and orbitofrontal cortex, respectively. These data and new preliminary data have built the foundation for the experiments described in this proposal. The hope is that we will have the same success in characterizing dysfunction in aging as we have had in the drug abuse field.
Roesch MR, Esber GR, Bryden DW, Cerri DH, Haney ZR, Schoenbaum G. Normal aging alters learning and attention-related teaching signals in basolateral amygdala. Journal of Neuroscience. 2012 Sep 19;32(38):13137-44.
Roesch MR, Bryden DW, Cerri DH, Haney ZR and Schoenbaum G. Willingness to wait and the encoding of time-discounted reward in the orbitofrontal cortex of aged rats. Journal of Neuroscience. 2012 Apr 18;32(16):5525-33
Pribut HJ, Vázquez D, Wei AD, Tennyson SS, Davis IR, Roesch MR, Li X.J. Overexpressing Histone Deacetylase 5 in Rat Dorsal Striatum Alters Reward-Guided Decision-Making and Associated Neural Encoding. Journal of Neurosci. 2021 Dec 8;41(49):10080-10090. doi: 10.1523/JNEUROSCI.0916-21.2021. Epub 2021 Oct 29.PMID: 34716230
Pribut HJ, Vázquez D, Brockett AT, Wei AD, Tennyson SS, Roesch MR. Prior cocaine exposure increases firing to immediate reward while attenuating cue and context signals related to reward value in the insula. Journal of Neuroscience. 2021 Apr 12:JN-RM-3025-20. PMID: 33849944
Conflict and Response Inhibition: In addition to reward-related functions, I have an interest in how the brain detects the competition between two competing behavioral actions (conflict; cognitive control) and how unwanted behavior is inhibited. My first contribution to this field was in graduate school. There, I published a paper with Kae Nakamura and Carl Olson showing that signals in anterior cingulate and supplemental eye field appear to better reflect the epiphenomenal manifestation of conflict (i.e., competing directional signals) as opposed to conflict monitoring. I continued examining this issue with Geoff Schoenbaum as post-doc, writing several papers, attempting to make the point that OFC does not encode conflict or response inhibition as suggested by many in the field. As a PI of my own lab I have continued to address this question head on showing that ACC and ABL are modulated by errors and attention during learning. Further we have recently developed a stop-signal task to characterize firing in DS, ACC, VTA, OFC, mPFC and Red Nucleus (RN). We have shown that activity in DS reflects the manifestation of conflict and the miscoding of directional signals, OFC signals conflict adaptation (i.e., increased executive control under heightened conflict), and that ACC and mPFC detects and monitors the degree of conflict. Further, we have shown that directional encoding in DS is dependent on ACC. Lastly, we have shown that prenatal nicotine exposure makes rats impulsive and disrupts firing of mPFC neurons during performance of this task.
Bryden DW and Roesch MR. Executive control signals in orbitofrontal cortex during response inhibition. Journal of Neuroscience, 35 (16), 6394-6400; (2015)
Bryden DW, Brockett AT, Blume E, Heatley K, Zhao A, and Roesch MR (2018). Single neurons in anterior cingulate cortex signal the need to change action during performance of a stop-change task that induces response competition. Cerebral Cortex. 2018. Feb 3. doi: 10.1093/cercor/bhy008.
Brockett AT, Tennyson SS, deBettencourt CA, Gaye F, Roesch MR (2020). Anterior cingulate cortex is necessary for adaptation of action plans. Proc Natl Acad Sci U S A. 2020 Mar 17;117(11):6196-6204. doi: 10.1073/pnas.1919303117. Epub 2020 Mar 4.
Brockett AT, Tennyson SS, deBettencourt CA, Kallmyer M, Roesch MR. Medial prefrontal cortex lesions disrupt prepotent action selection signals in dorsomedial striatum. Curr Biol. 2022 Aug 8;32(15):3276-3287.e3. doi: 10.1016/j.cub.2022.06.025. Epub 2022 Jul 7.PMID: 35803273
Social recognition of reward and distress: Understanding how the well-being of others affects our behavior is critical, but vastly understudied. Several disorders are characterized by an inability to recognize others’ mental states (e.g., psychopathy, autism). Human imaging work has provided clues regarding core structures involved in this process, including the amygdala, anterior cingulate (ACC), striatum, and orbitofrontal cortex; however, detailed work in animals at the single-unit and neurotransmitter level has not yet occurred. The computational basis of this process is critical for understanding fundamental mechanisms that are necessary and sufficient for these behaviors to occur. Given the current absence of work modeling these mechanisms in animals at the neuronal level we have started to address this issue in the lab. Our first contribution to this field was to show for the first time that DA release is modulated by delivery of reward to a conspecific. Our data show that animals display a mixture of affective states during observation of conspecific reward, first exhibiting increases in appetitive calls (50 kHz), then exhibiting increases in aversive calls (22 kHz). Like ultrasonic vocalizations (USVs), DA signals were modulated by delivery of reward to the conspecific, which mapped onto the emotional state associated with the conspecific receiving reward. Our results demonstrated the positive and negative states associated with conspecific reward delivery modulate DA signals related to learning in social situations. Following up on those results, we have also shown that though we find clear signs of ‘empathy’ and ‘pro-social’ behavior in rats, they are often ‘self-interested’; their behavior and DA signals were modulated by potential reward and shock that occurred directly to them as opposed to the conspecific, reflecting subjective rather than objective value.
Most recently, we have turned our sights to ACC. Neural recording studies have shown that neurons in ACC are modulated by reward or shock when delivered to a conspecific and when experienced first-hand. Since previous studies do not vary reward and shock within the same experiment, it has been unclear whether the observed activity reflects how much attention is being paid to outcomes delivered to a conspecific or the valence associated with those stimuli. To address this issue, we recorded from ACC as rats performed a Pavlovian task that predicted whether reward, shock, or nothing would be delivered to the rat being recorded from or a conspecific located in the opposite chamber. Consistent with previous reports, we found that the firing of ACC neurons was modulated by aversive stimuli delivered to the recording rat and their conspecific. Activity of some of these neurons genuinely reflected outcome identity (i.e., reward or shock), however the population of neurons as a whole responded similarly for both reward and shock, as well as for cues that predicted their occurrence (i.e., reward >neutral and shock > neutral; attention). These results suggest that ACC can process information about outcomes (i.e., identity, recipient) in the service of promoting attention in some social contexts.
Kashtelyan V, Lichtenberg NT, Chen ML, Cheer JF, and Roesch MR. (2014) Observation of reward delivery to a conspecific modulates dopamine release in ventral striatum. Current Biology 24 (21), 2564-2568.
Lichtenberg NT, Lee B, Kashtelyan V, Chappa BS, Girma HT, Green EA, Kantor S, Lagowala DA, Myers MA, Potemri D, Pecukonis MG, Tesfay RT, Walters MS, Zhao AC, Blair RJR, Cheer JF, Roesch MR (2018). Elife. 2018 Nov 28;7. pii: e38090. doi: 10.7554/eLife.38090.
Schneider KN, Sciarillo XA, Nudelman JL, Cheer JF, Roesch MR. Anterior Cingulate Cortex Signals Attention in a Social Paradigm that Manipulates Reward and Shock. Current Biology. 2020 Oct 5;30(19):3724-3735.e2. doi: 10.1016/j.cub.2020.07.039. Epub 2020 Aug 6.PMID: 32763169
Vázquez D, Schneider KN, Roesch MR. Neural signals implicated in the processing of appetitive and aversive events in social and non-social contexts. Front Syst Neurosci. 2022 Aug 3;16:926388. doi: 10.3389/fnsys.2022.926388. eCollection 2022.PMID: 35993086
The use of molecular container compounds (MCCs) to sequester drugs of abuse – Over 1.25 million emergency room visits were reported in 2011 due to abuse of illicit drugs in the US. Of the 1.25 million visits, opiates (heroin, fentanyl, prescription opiates), (meth)amphetamines, and PCP accounted for roughly 40% of cases. Critically, with the exception of opiates, amphetamines and dissociatives, like PCP, lack any viable countermeasure to prevent death by overdose. Even with opiates, for which naloxone has been vital in preventing overdose-related deaths, the increase in fentanyl-related overdoses has steadily risen in recent years. This is particularly problematic as the ability of naloxone to counteract fentanyl is substantially lower, often requiring more doses of naloxone and greater patient care. This work has investigated the ability of MCCs to act as a countermeasure for a variety of drugs of abuse including, fentanyl, methamphetamine, and PCP. We have synthesized several novel MCCs, performed toxicology and mutagenicity assays, and tested their abilities to reverse drug-induced hyperlocomotion in mice performing an autoshaping task. This work has been critical in identifying sequestration agents that can be nominated toward US FDA investigational new drug status.
Adam T. Brockett, Weijian Xue, Chun-Lin Deng, Canjia Zhai, Michael Shuster, David King, Shivangi Rastogi, Volker Briken, Matthew R. Roesch, Lyle Isaacs “Pillar[6]MaxQ: A Potent Supramolecular Host for In Vivo Sequestration of Methamphetamine and Fentanyl” CHEM 2023, ASAP. DOI: https://doi.org/10.1016/j.chempr.2022.11.019.
DiMaggio D, Brockett AT, Shuster M, Murkli S, Zhai C, King D, O'Dowd B, Cheng M, Brady K, Briken V, Roesch MR, Isaacs L. Anthracene-Walled Acyclic CB[n] Receptors: in vitro and in vivo Binding Properties toward Drugs of Abuse. ChemMedChem. 2022 May 18;17(10):e202200046. PubMed Central PMCID: PMC9119912.
Brockett AT, Deng C, Shuster M, Perera S, DiMaggio D, Cheng M, Murkli S, Briken V, Roesch MR, Isaacs L. In Vitro and In Vivo Sequestration of Methamphetamine by a Sulfated Acyclic CB[n]-Type Receptor. Chemistry. 2021 Dec 9;27(69):17476-17486. PubMed Central PMCID: PMC8665056.
Murkli S, Klemm J, Brockett AT, Shuster M, Briken V, Roesch MR, Isaacs L. In Vitro and In Vivo Sequestration of Phencyclidine by Me4 Cucurbit[8]uril*. Chemistry. 2021 Feb 10;27(9):3098-3105. PubMed Central PMCID: PMC7902406.
If you are interested in joining the lab please contact Matt Roesch at mroesch@umd.edu.
Please visit our website at...
http://roeschlab.wixsite.com/roeschlab
Degrees
-
PhDDepartment of Neuroscience and Center for the Neural Basis of Cognition, Univesity of Pittsburgh
Current Students
-
-
Grad Advisee Profile
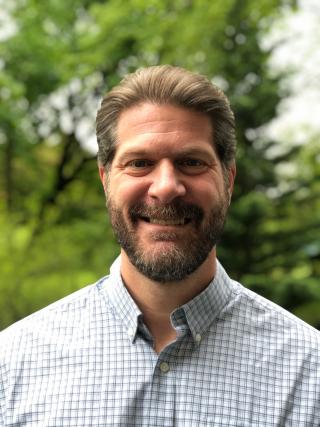